Diagnosing and treating cancer is less straightforward than it once seemed to be. For example, time was that cancer of the breast was breast cancer, for which doctors had only a limited treatment arsenal to choose from. However, as ongoing research sheds new light on the matter, the picture has become more complex. A case in point is a study published in 2012, led by Professor Carlos Caldas, who has held the Chair of Cancer Medicine at the University of Cambridge since 2002. Dr. Caldas, who also heads the Breast Cancer Functional Genomics Laboratory at the Cancer Research UK Cambridge Research Institute, showed that the disease category known collectively as “breast cancer” actually covered at least ten different diseases, and that genome sequencing shows that even one type of breast cancer can differ between individuals.
Such enhancements in the state of scientific knowledge underscore the complexity of cancer biology, but also hold forth the promise of drug therapy that can be tailored to an individual as an alternative to the powerful, but blunt, instrument that is chemotherapy, which not only attacks the cancer tumor, but also attacks several of the body’s other functions, making patients very ill. The aim of a new generation of cancer drugs is to make the tumor sick, but not the patient.
“But telling if a patient is sick is easy; telling if the tumor is sick is more challenging. Conventionally, one assesses whether a tumor is responding to treatment by looking for evidence of shrinkage,” explains Professor Kevin Brindle from the Cancer Research UK (CRUK) Cambridge Institute in a U. of Cambridge release, “but that can take weeks or months. And monitoring tumor size doesn’t necessarily indicate whether it is responding well to treatment.”
Dr. Brindle notes that brain tumors, for example, can continue to grow even when a treatment is working, noting that “The thing is that a tumor is not just tumor cells. There are lots of other cells in there, too.”
Molecular imaging in cancer
The primary aim of the Brindle Group laboratory at the Cancer Research UK Cambridge Institute (CRUK) is to develop clinically applicable imaging methods that can be used to detect early tumor responses to treatment that could be used in early stage clinical trials of new drugs to get an indication of efficacy, and subsequently, in the clinic, to guide therapy in individual patients.
As noted above, even patients with similar tumor types may show substantially different responses to the same therapy, which means that development of new treatments would benefit from availability of imaging methods that would allow an early assessment of treatment response in individual patients, and in turn timely selection of the most effective treatment for a specific patient (Brindle, Nat. Rev. Cancer 2008; 8: 1).
Consequently, the primary focus of the Brindle Lab’s work is early detection of treatment response with the aim of developing imaging methods that could be used in early phase clinical trials to get an indication of drug efficacy, and subsequently in the clinic to guide treatment in individual patients (Brindle, Nat Rev Cancer 2008; 8: 1). The lab scientists have also started to work on the more challenging problem of early detection and the development of imaging methods that potentially could be used for patient screening.
Through a partnership with GE Healthcare, the Brindle Lab researchers are developing a technology called “nuclear spin hyperpolarization” as a novel tool for molecular imaging. They note that nuclear spin polarization offers enormous gains in sensitivity, as much as 10,000x, a level of magnification that makes it possible not only to image the distribution of isotopically-labelled cellular metabolites, but also their enzymatic transformation into other species. “This provides us with potentially more sensitive ways to detect tumor responses to treatment and, with some substrates, is providing new insights into cellular metabolism in vivo.”
[adrotate group=”1″]
For example, using the reduced and oxidized forms of vitamin C (ascorbic acid), which have been labelled with hyperpolarised 13C, we are able to image tumor redox state in vivo,” explains Dr. Brindle. Since aberrant glycosylation is a hallmark of cancer, he says, “We are developing a novel molecular imaging platform for the non-invasive assessment of tumor glycosylation, in which sugar analogues are incorporated metabolically by tumor cells in vivo and subsequently detected by a highly selective chemical reaction (click chemistry) with a reporter probe that has been labelled with an imaging agent. This methodology could provide new insights into tumor cell proliferation, response to therapy, and metastasis.”
Imaging Metabolism With Hyperpolarised 13c-labelled Cell Substrates
An alternative technique that doesn’t expose the patient to ionizing radiation is magnetic resonance imaging (MRI), which provides excellent soft tissue images of, for instance, tumors — a technique that works by imaging distribution and MR properties of tissue water protons, which are very abundant (60 70 M in tissues), and relies on the interaction of strong magnetic fields with a property of atomic nuclei known as spin. The proton spins in water molecules and align in magnetic fields, like tiny bar magnets. By looking at how these spins differ in the presence of magnetic field gradients applied across the body, scientists are able to build up three-dimensional images of tissues.
“However, we can also use MR to detect metabolites in vivo. The problem is that these molecules are present at ~10,000 lower concentration than the protons in tissue water, which makes them hard to detect and almost impossible to image, except at very low resolution,” Dr. Brindle observes. “We have been collaborating with GE Healthcare in the development of a technique, termed hyperpolarization, that increases sensitivity in the MRI experiment by more than 10,000. With this technique we inject a hyperpolarised 13C-labelled molecule and now have sufficient sensitivity to image its distribution in the body and the distribution of the metabolites produced from it. Previously we had shown we can detect early response to chemotherapeutic drugs by monitoring decreased tumor utilization of one cell metabolite, pyruvate, and then detect subsequent cell death by watching the increased metabolism of another metabolite, fumarate. We are taking these forward in a clinical trial, which is due to start in 2014, to detect treatment response in lymphoma, glioma and breast cancer patients. A clinical device for hyperpolarising 13C-labelled pyruvate and fumarate was installed in the Department of Radiology in Addenbrooke’s Hospital earlier this year.”
In the 1970s, scientists realized that it was possible to use MR spectroscopy to observe signals from metabolites such as glucose inside cells, and for some time now, oncologists have been interested in the potential of imaging aspects of tumor biology for providing much earlier indication of the effect of treatment. Positron emission tomography (PET) can be used for this purpose. The patient is injected with a form (or analog) of glucose labelled with a radioactive isotope. Tumors feed on the analogue and the isotope allows doctors to see where the tumor is.
Scientists also discovered that enriching metabolites with a naturally occurring isotope of carbon known as carbon-13 to help them measure how these metabolites are used by tissues. However, carbon-13 nuclei are even less sensitive to detection by MRI than protons, so the signals are boosted using a machine developed by GE Healthcare, called a hyperpolariser, which lines up a large proportion of the carbon-13 spins before injection into the patient.
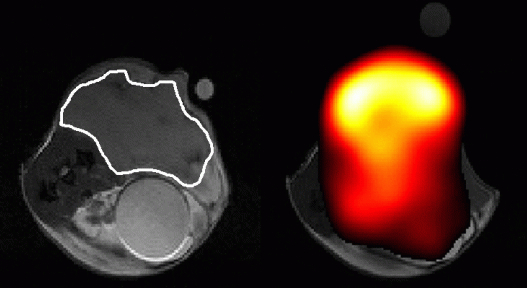
“Tumors eat and breathe,” says Dr. Brindle. “If you make them sick, they don’t eat as much and the concentration of some cell metabolites can go down.”
In 2006, Cambridge was among the first to show that this approach could be used to monitor whether a cancer therapy was effective or not. Combined with the latest genome sequencing techniques, the technology they developed could become a powerful way of implementing personalized medicine, and moreover, because there are no radioactive isotopes are involved, a patient can be scanned safely multiple times.
Because of the underlying genetics of the tumor, not all patients respond in the same way, “but if you sequence the DNA in the tumor, you can select drugs that might work for that individual. Using hyperpolarization and MRI, we can potentially tell whether the drug is working within a few hours of starting treatment. If it’s working you continue, if not you change the treatment,” says Dr. Brindle.
The challenge, he says, has been how to deliver the carbon-13 to the patient. The metabolite must be cooled down to almost absolute zero (273C), polarized, warmed up rapidly, passed into the MRI room and injected into the patient. And as the polarization of the carbon-13 nuclei has a half-life of only 3040 seconds, this all must be executed very rapidly. However, that problem has largely been solved and, with funding from the Wellcome Trust and CRUK, Dr. Brindle and his colleagues will begin trials of the technique with cancer patients at Addenbrooke’s Hospital — a world-renowned teaching hospital in Cambridge, England, with strong University of Cambridge affiliations. If proven successful in real-world applications it could revolutionize both evaluation of new drugs and ultimately — and most importantly — treatment of patients.
“Some people have been skeptical about whether we could ever get a strong enough signal. Im sure we will. But will we be able to do something that is clinically meaningful, that is going to change clinical practice? Thats the big question we hope to answer in the coming years,” Dr. Brindle affirms.
Lighting Up The Body
Looking ahead, the scientists observe that in many ways, light microscopy is an even better imaging technique than MRI and PET for studying the nature of biological materials. Light Microscopy provides higher resolution and higher specificity as fluorescent markers can be used to highlight specific cancer cells and molecules in cells and tissues.
However, Dr. Stefanie Reichelt, Head of at the Cancer Research UK Cambridge Institute, says there’s an obvious caveat. Ergo: light doesn’t penetrate tissue, so we can’t see deep beneath the skin using Light Microscopy. Dr. Reichelt’s research goal is development of advanced non-linear imaging including multi-photon fluorescence imaging with fluorescence lifetime information as well as second harmonic imaging (SHG) for tumor detection in live cells and biopsies. She and her colleagues are working on ways to correlate light microscopy with Kevin Brindle’s medical imaging techniques, and report that one such technique that shows promise for bridging the gap is “light sheet microscopy” — a fluorescence microscopy technique with an intermediate optical resolution.
The scientists explain that a thin slice of the sample is illuminated perpendicularly to the direction of observation, thus reducing photo damage and allowing high-speed, high-resolution, three-dimensional imaging of live animals and tissues.
“The key for us is to be able to image whole biopsy samples or tumors rapidly and at a high level of detail,” says Dr. Reichelt who is also exploring new techniques such as Coherent Anti-Raman Stokes, which uses the nuclear vibrations of chemical bonds in molecules. “This can provide a highly specific but label-free imaging contrast — a capability that will allow investigation of unlabeled live tissues from tumor biopsies with high specificity.”
See more at:
http://www.cam.ac.uk/research/features/watching-the-death-throes-of-tumors#.dpuf
Sources:
The University of Cambridge
Cancer Research UK Cambridge Institute
Image Credits:
Cancer Research UK Cambridge Institute
Kevin Brindle
Addenbrooke’s Hospital